Aerodynamics: Definition, Function, Formula, Facts
Jim Goodrich • • Reading time: 14 min
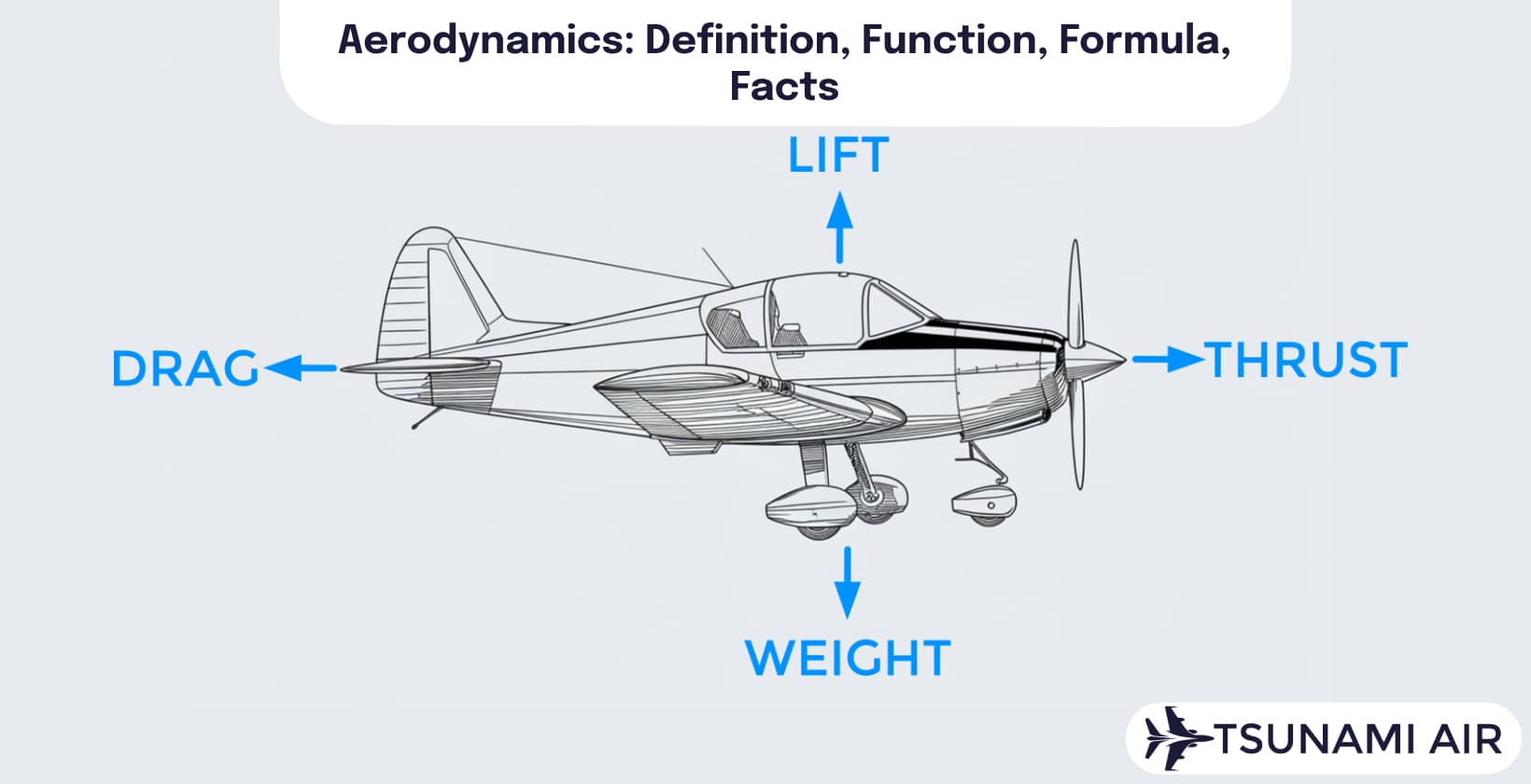
Aerodynamics is the study of how air interacts with moving objects in the design of aircraft and vehicles. Aerodynamics involves forces like lift and drag, which are influenced by factors including shape, velocity, and pressure. Aerodynamics utilizes formulas and coefficients to calculate air resistance and fluid dynamics around airfoils and wings. Learn about aerodynamics' principles, applications, and facts to understand its function in aviation and engineering.
Aerodynamics of a plane involves four forces: lift, weight, thrust, and drag. Lift opposes weight and is generated by wings through air motion. Wings create lift force through faster airflow over their curved top surface, resulting in reduced pressure above the wings. Air properties including density, viscosity, pressure, and temperature impact aerodynamic performance. Density and viscosity changes alter overall aerodynamic behavior, affecting lift generation, drag forces, and thrust efficiency.
The Continuity Equation in aerodynamics, expressed as A1V1 = A2V2, relates area and velocity in fluid flow. The Drag Equation, FD = 1/2 ρv²CDA, calculates drag force based on fluid density, velocity, drag coefficient, and reference area. Lift Equation, L = 1/2 ρv²CLA, determines lift force using lift coefficient, density, velocity, and wing area. The Navier-Stokes Equations describe fluid motion by combining viscosity, velocity field, and pressure distribution.
Airfoil shape enhances lift through camber and angle of attack adjustments. Boundary layer dynamics affect drag and lift, with flow separation increasing drag. Computational fluid dynamics and wind tunnel testing optimize aerodynamic designs. Aerodynamic principles apply to aviation, automotive design, and structural engineering for wind resistance. Sir Isaac Newton developed a theory of air resistance, while Daniel Bernoulli formulated the principle relating pressure and velocity in fluids. Aerodynamics forms a subset of fluid dynamics, focusing on air behavior and interaction with solid objects.
What is the definition of aerodynamics?
Aerodynamics is the branch of physics that studies the motion of air and its interaction with objects, focusing on the forces acting upon bodies moving through fluids. Aerodynamics encompasses the study of lift, drag, and thrust, which are crucial for understanding how aircraft fly. Aerodynamics utilizes computer simulations and wind tunnel experimentation to refine designs and address issues like compressible flow and turbulence. Sir Isaac Newton and Daniel Bernoulli made contributions to aerodynamics, with Newton developing a theory of air resistance and Bernoulli formulating the principle relating pressure and velocity in fluids. Aerodynamics affects the design of aircraft, cars, and other vehicles that move through air, refining speed and fuel efficiency.
Aerodynamics forms a subset of fluid dynamics, focusing on the behavior of fluids like air. The science examines air motion and interaction with solid objects, emphasizing the properties and compressibility of gases. Lift generation produces an upward force that opposes an object's weight through pressure differences on surfaces. Drag force resists motion through air, requiring thrust to overcome this resistance. Pressure and force interactions are important to understanding aerodynamic principles, with airflow patterns and streamlines playing key roles in determining object behavior.
Aerofoil geometry influences lift generation through its curved upper surface shape. Object shape affects aerodynamic performance, with certain designs minimizing drag for smoother motion through the air medium. Aerodynamics predicts moving objects' trajectories by managing lift and drag forces, enabling control of flight paths. Turbulence instability causes pressure and velocity variations, increasing drag and reducing motion efficiency. Aerodynamics concepts involve fluid dynamics behavior and flow characteristics, including boundary layer effects and flow separation phenomena. Aerodynamics principles optimize vehicle and aircraft performance by reducing drag resistance and bettering lift generation.
What is load factor in aerodynamics?
Load factor in aerodynamics is a ratio comparing aircraft lift to weight. Load factor represents stress on aircraft structure during maneuvers, expressed as n = L/W. Load factor affects stalling speed and is fundamental for pilot decision-making and aircraft design. Civil aviation authorities specify load factor limits for aircraft categories.
Load factor represents the relationship between lift and weight in aircraft performance. Lift magnitude includes contributions from wings, fuselage, and tailplane, while weight force acts on aircraft mass. G-force acceleration relates to load factor expression, with a dimensionless value expressed in g units. Altitude affects load factor through air density changes, impacting aerodynamic forces on the aircraft.
Bank angle and roll influence load factor during turns. A 60-degree bank angle results in a load factor of 2, doubling the weight of the aircraft. Pressure distribution in aerodynamics plays a part in determining lift force and consequently load factor. Aerodynamics and fluid dynamics principles govern the interactions between air molecules and aircraft surfaces, affecting load factor.
Aircraft design incorporates load factor limits for categories to guarantee structural integrity. Transport category aircraft have load factor limits of +2.5g ( +24.5 ft/s²) to -1g (-9.8 m/s²), while aerobatic aircraft can withstand up to +6g ( +58.8 ft/s²) to -3g ( -29.4 m/s²). Performance calculations rely on load factor data to determine aircraft capabilities and limitations. Flight envelope limitations are tied to load factor, defining the safe operating range for speed, altitude, and maneuvers. Load factor increases with maneuvers requiring more lift, imposing greater stress on the airframe and approaching structural limits.
How do the aerodynamics of a plane work?
The aerodynamics of a plane work through four forces: lift, weight, thrust, and drag. Lift is generated by the wing's curved upper surface and flat bottom. Air flowing over the wing creates lower pressure above, producing upward force. Thrust from engines counteracts drag, allowing steady flight.
Airplane wing shape, size, and camber influence lift generation. Wings with greater surface area produce more lift. Increased wing camber enhances lift by accelerating airflow over the top surface. Lift magnitude depends on factors like airspeed, air density, and angle of attack. The lift coefficient measures how an airfoil generates lift. Bernoulli's Principle explains the pressure difference created by faster airflow over the wing top. Airfoil properties like curvature, chord length, and thickness affect lift production. Angle of attack, the angle between the wing and airflow, directly impacts lift generation.
Drag opposes airplane motion through the air. Drag magnitude increases with airspeed and air density. The drag coefficient quantifies an object's resistance to air flow. Drag components include friction drag from air viscosity and pressure drag from air pressure differences. Boundary layer characteristics like thickness and flow type (laminar or turbulent) influence drag. Thrust counteracts drag to maintain forward motion. Engine power output determines thrust magnitude. Thrust direction depends on engine mounting on the aircraft. Weight, the gravitational force on the airplane's mass, opposes lift.
Air pressure distribution around the wing is decisive for lift generation. Lower pressure above and higher pressure below creates upward force. Air density affects lift and drag forces on planes. Newton's Third Law applies to aerodynamic forces in flight. Thrust acts as the action force propelling the plane forward. Drag serves as the reaction force opposing motion. Lift acts against weight to achieve equilibrium in steady flight. Control surfaces like ailerons, elevators, and rudders allow pilots to adjust airflow and control plane orientation. These surfaces' mobility, effectiveness, and adjustment are vital for maintaining flight.
What are the principles of aerodynamics?
The principles of aerodynamics involve four forces: lift, weight, thrust, and drag. Lift opposes weight, enabling flight. Thrust propels aircraft, overcoming drag. Air density and velocity affect these forces. Aerodynamic principles guide aircraft design, balancing forces for efficient flight.
Lift force results from pressure differences over wing surfaces. Airfoil profile generates lift through unequal air pressure distribution above and below the wing. Weight force pulls aircraft downward due to Earth's gravity. Aircraft mass determines the magnitude of weight force acting on the vehicle. Drag aerodynamic resistance opposes aircraft motion through air. Flow separation increases drag on aircraft, at high angles of attack. Thrust propulsive force propels aircraft forward through air. Engines or propellers generate thrust by accelerating air masses, creating a momentum change.
Airfoil geometry affects lift and drag characteristics of wings. Airfoil camber influences pressure distribution over wing surfaces, with increased camber producing higher lift. Angle of attack incidence impacts lift generation on wings. Angle of attack orientation alters airflow patterns around airfoils, affecting pressure distribution and lift production.
Bernoulli's principle explains pressure gradient creation for lift generation. Faster-moving air over the upper wing surface creates lower pressure compared to slower air below. Newton's laws of motion govern aerodynamic behavior of aircraft. Newton's first law describes inertia of objects in motion, while the second law relates force to mass and acceleration. Newton's law explains action-reaction forces in flight, namely lift opposing weight.
What is the aerodynamics formula?
The aerodynamics formula is expressed as A1V1 = A2V2, representing the continuity equation. This formula relates area and velocity in fluid flow, stating that the product of cross-sectional area and velocity remains constant in incompressible flow systems.
The Drag Equation formula is expressed as FD = 1/2 ρv²CDA. FD represents the drag force, ρ is fluid density, v is velocity, CD is the drag coefficient, and A is the reference area. The Aerodynamic Force Equation builds on this concept, incorporating dynamic pressure and force coefficients to calculate overall aerodynamic forces on an object.
Navier-Stokes Equations are important in fluid dynamics, describing fluid motion by combining viscosity, velocity field, and pressure distribution. These equations enforce momentum conservation and are fundamental for flow analysis. Bernoulli's Equation, given as p + 1/2 ρv² + ρgh = constant, relates pressure, velocity, and energy conservation for inviscid, incompressible flows.
The Lift Equation, L = 1/2 ρv²CLA, calculates lift force based on the lift coefficient (CL), density (ρ), velocity (v), and wing area (A). Euler Equations describe inviscid flow, focusing on momentum conservation and pressure distribution without accounting for viscosity effects. Engineers and scientists apply these formulas to design aircraft, analyze flow scenarios, and solve aerodynamics problems through computational dynamics.
What are some examples of aerodynamics?
Examples of aerodynamics include airplane wings generating lift, rocket propulsion overcoming drag during launch, bird flight utilizing wing shape for lift, kite flying demonstrating lift and drag effects, and car body designs reducing air resistance for efficiency and stability.
Some examples of aerodynamics are provided in the list below.
- Airplane aerodynamics: Wings generate lift through a cambered airfoil, and streamlined fuselages reduce drag.
- Helicopter aerodynamics: Rotor systems generate lift and thrust, with blade design impacting performance.
- Rocket aerodynamics: Fin stability and nose cone shapes minimize drag for varied speed regimes.
- Missile aerodynamics: Fins for maneuverability and nose designs for speed and radar absorption.
- Recreational aerodynamics: Kite and glider designs utilize airfoil principles for lift and efficiency.
- Automotive aerodynamics: Car bodies reduce drag and spoilers increase downforce for stability.
- Bird aerodynamics: Wing aspect ratios and feather structure enhance lift and efficiency.
- UAV aerodynamics: Drone propellers generate thrust, and frame designs focus on reduced drag.
- Parachute aerodynamics: Canopy designs manage controlled descent through drag generation.
The airplane wing generates lift through its curved shape, creating a pressure difference between the upper and lower surfaces. Airplane wings have a cambered airfoil design, with a curved upper surface to accelerate airflow. The airplane fuselage is streamlined to minimize drag, featuring a tapered shape towards the rear. Streamlined fuselages reduce air resistance by up to 65% compared to non-aerodynamic shapes. The airplane tail provides stability and control, consisting of vertical and horizontal stabilizers. Tail configurations vary, with T-tails offering refined stability at high angles of attack.
Helicopter aerodynamics centers around the rotor system. Helicopter rotors generate both lift and thrust through blade rotation. Rotor blades are airfoils, similar to airplane wings, with designed twist and taper. Blade angle of attack is pivotal, ranging from 2 to 12 degrees for good performance. Helicopter blade shape and angle impact lift generation and power efficiency.
Rocket and missile aerodynamics focus on stability and drag reduction. Rocket fins provide stability by creating drag at the rear of the vehicle. Fins are angled at 0.5 to 1 degree for performance. The rocket nose cone shape minimizes drag, with designs for subsonic and supersonic speeds. Supersonic rockets use nose cones with half-angles of 10 to 15 degrees. Missile fins enhance maneuverability. Missile nose cones are designed for high-speed flight, incorporating radar-absorbing materials.
Recreational and sports aerodynamics utilize aerodynamics principles. Kite sails act as airfoils, generating lift through their curved shape. Kite airfoils have a camber of 8-12% for lift. Glider wings maximize lift-to-drag ratios, featuring aspect ratios of 20 or more. Glider streamlining reduces drag, with fuselages and designed wing-body junctions.
Automotive aerodynamics focuses on drag reduction and stability. Car bodies are designed to minimize air resistance, with designs achieving drag coefficients low as 0.22. Car spoilers increase downforce by creating low-pressure areas, refining traction at high speeds. Spoilers can increase downforce by up to 100 pounds (45.36 kilograms) at highway speeds.
Bird wings have evolved for lift generation, with aspect ratios varying from 4 in sparrows to over 9 in albatrosses. Bird feathers contribute to aerodynamic efficiency through their structure and ability to adjust during flight.
In Unmanned Aerial Vehicle (UAV), drone propellers generate thrust through rotation, with speeds ranging from 5,000 to 15,000 RPM. Drone frames are designed for reduced drag while maintaining stability, featuring materials and shapes that enhance aerodynamics.
Parachute aerodynamics focuses on controlled descent. Parachute canopies create drag through their large surface area, with descent rates of 10-20 feet per second (3.05-6.10 meters per second). Canopy designs vary, with some featuring vents or slots to boost stability and control.
What are the facts about aerodynamics?
Facts about aerodynamics include that it is a field that studies air interactions with moving objects. Different forces govern object motion through air. Lift, weight, thrust, and drag are forces in flight. Object shape influences air interaction efficiency.
The facts about aerodynamics are outlined below.
- Aerodynamics studies interactions between air and moving objects.
- Forces in aerodynamics include lift, weight, thrust, and drag, which govern object motion.
- Aerodynamic principles explain airplane flight, car performance, and rocket propulsion.
- Aerodynamic efficiency is influenced by object shape.
- Aerodynamics explains how airplanes fly by balancing lift, weight, thrust, and drag.
- Lift generation relies on air motion around wings, with wing shape being decisive.
- Drag in aerodynamics resists forward motion and is shape-dependent.
- Thrust in aerodynamics propels aircraft to counteract drag.
- Air properties including density, viscosity, and pressure affect aerodynamics and performance.
- Navier-Stokes equations describe fluid motion in aerodynamics.
- Reynolds number predicts flow regimes and turbulence in aerodynamics.
- Bernoulli's principle explains pressure-velocity relationships in lift generation in aerodynamics.
- Airfoil shape in aerodynamics enhances lift through adjustments in camber and angle of attack.
- Boundary layer dynamics affect drag and lift in aerodynamics, influenced by flow separation.
- Angle of attack impacts lift and drag in aerodynamics and aircraft performance.
- Computational fluid dynamics are used to optimize aerodynamic designs.
- Aerodynamics applications are integral to aviation, automotive design, and structural engineering.
Aerodynamics explains how airplanes fly by balancing four forces: lift, weight, thrust, and drag. Lift opposes weight and is generated by air motion around wings. Thrust opposes drag and is provided by engines or propellers. Wing shape creates lift force through faster airflow over the curved top surface. Reduced pressure above the wing generates lift to counteract the weight force. Drag resists forward motion and is influenced by object shape. Thrust propels the aircraft to overcome drag.
Air density impacts lift generation and drag forces. Air viscosity influences boundary layer formation and flow separation. Air pressure affects lift production and thrust efficiency. Air temperature changes density and viscosity, altering overall aerodynamic behavior. Navier-Stokes equations describe fluid motion and model aerodynamic flows. Reynolds number determines flow regime and predicts turbulence effects.
Bernoulli's principle explains the pressure-velocity relationship for lift generation. Angle of attack changes lift and drag forces, impacting aircraft performance. Computational fluid dynamics and wind tunnel testing are used to optimize aerodynamic designs. Aerodynamic principles apply to fields including aviation, automotive design, and structural engineering for wind resistance.